Steels solidify as solid solutions. The face-centered cubic lattice structure with the embedded carbon atom is called austenite.
Introduction
In principle, steels are binary systems consisting of the host element iron and the alloying element carbon with a maximum content of 2 % (above 2% carbon, the iron-carbon alloy is called cast iron!). The carbon provides the necessary strength and hardness because iron alone would be too soft as a construction material. In order to be able to produce steels according to these different requirements (high hardness or high strength, or a compromise of both), a deeper understanding of the alloy system iron/carbon is required.
Steel is an alloy of iron and carbon! With a carbon content of more than 2 % one speaks of cast iron!
In contrast to the binary systems previously considered, phase transformation does not only take place during solidification. Iron also shows an allotropy (polymorphism), i.e. depending on temperature iron exists in different lattice structures. In the solid state, these cause further phase transformations. Therefore, the phase diagram of the iron/carbon alloy system is somewhat more complex.
In order to understand the microstructural processes inside a steel, it makes sense to first take a closer look at the microstructure formation of pure iron. For this reason, the cooling curve of iron is discussed in more detail in the following section.
Microstructure formation of soft iron
In the following, the cooling curve of pure iron will be examined in more detail. Since pure iron is relatively soft in the solidified state, it is also called soft iron.
The cooling curve of pure iron (Fe) has a series of thermal arrests at which different processes take place in the microstructure. The first thermal arrest is at the solidification temperature of 1536 °C. At this point the melt crystallizes in a body-centered cubic lattice structure (bcc). In this state the iron is also called \(\delta\)-iron (\(\delta\)-Fe). Note that the entire microstructure of \(\delta\)-iron is already completely solidified. Thus, all further phase transformations finally take place in the already solidified state!
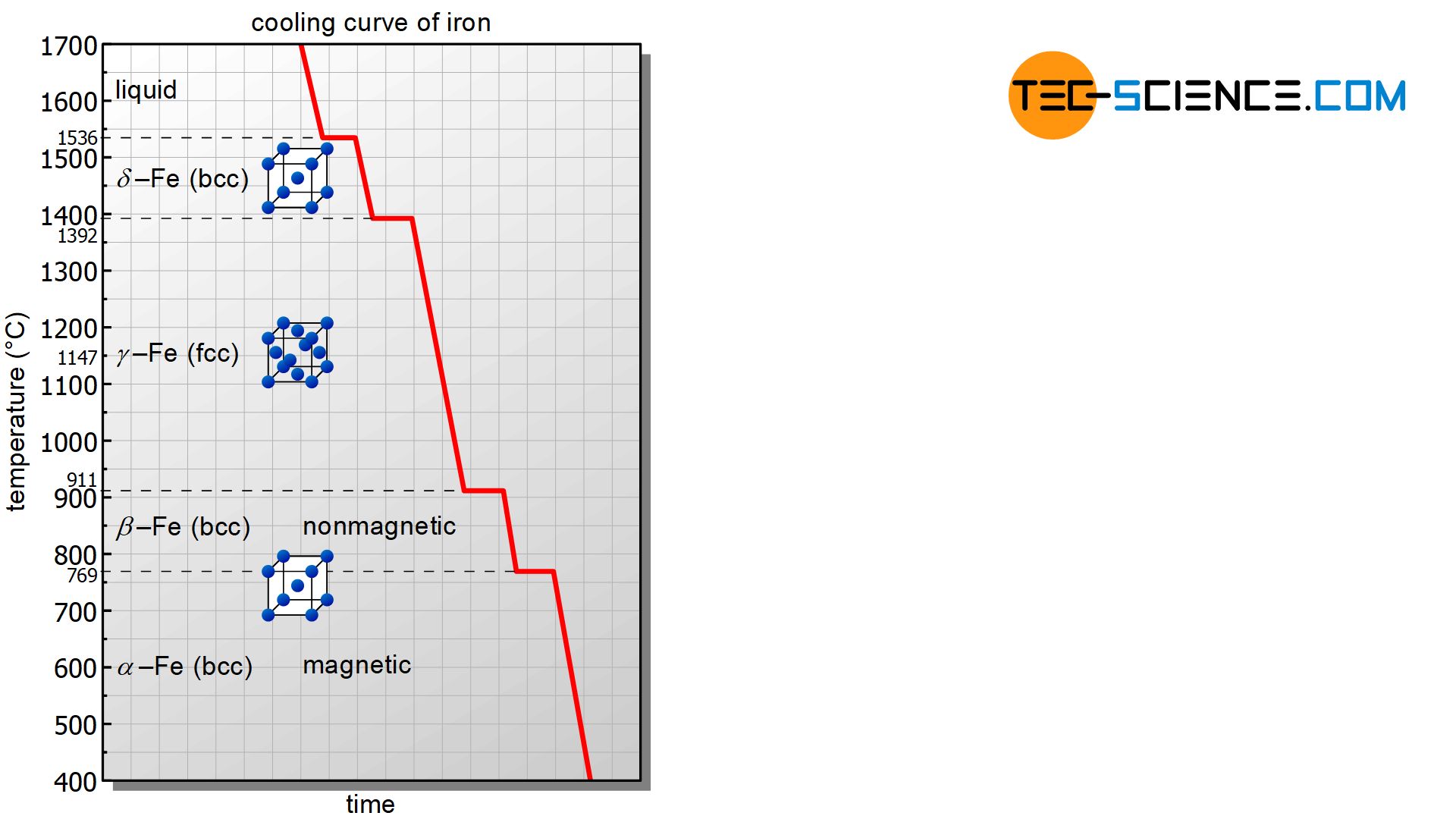
At a temperature of 1392 °C, the body-centered cubic \(\delta\)-iron transforms into the face-centered cubic structure (fcc) at a constant temperature. In this lattice modification the iron is also called \(\gamma\)-iron. Since the atomic structure and thus the binding energies change during a lattice transformation, this is also associated with an energy conversion. Therefore, the lattice structure changes at a constant temperature (thermal arrest)!
A further lattice transformation finally takes place at 911 °C. At this temperature, the face-centered cubic iron transforms back into the body-centered cubic structure. In this form the iron is also called (\beta\)-iron.
A last thermal arrest finally occurs at a temperature of 769 °C. However, this is not due to a lattice transformation! The reason for the thermal arrest is a quantum mechanical effect, which is responsible for the fact that the iron is magnetic below this temperature and not above! This temperature is also called Curie temperature (apart from iron, only the elements cobalt and nickel are ferromagnetic at room temperature). The magnetic state of iron with its body-centered cubic lattice structure is also called (\alpha\)-iron.
The Curie temperature is the temperature at which a ferromagnetic material loses its magnetic properties!
The micrograph below shows soft iron (\(\alpha\)-iron) in an almost carbon-free state. The iron grains (white areas) and silicate inclusions (dark spots) can be seen.
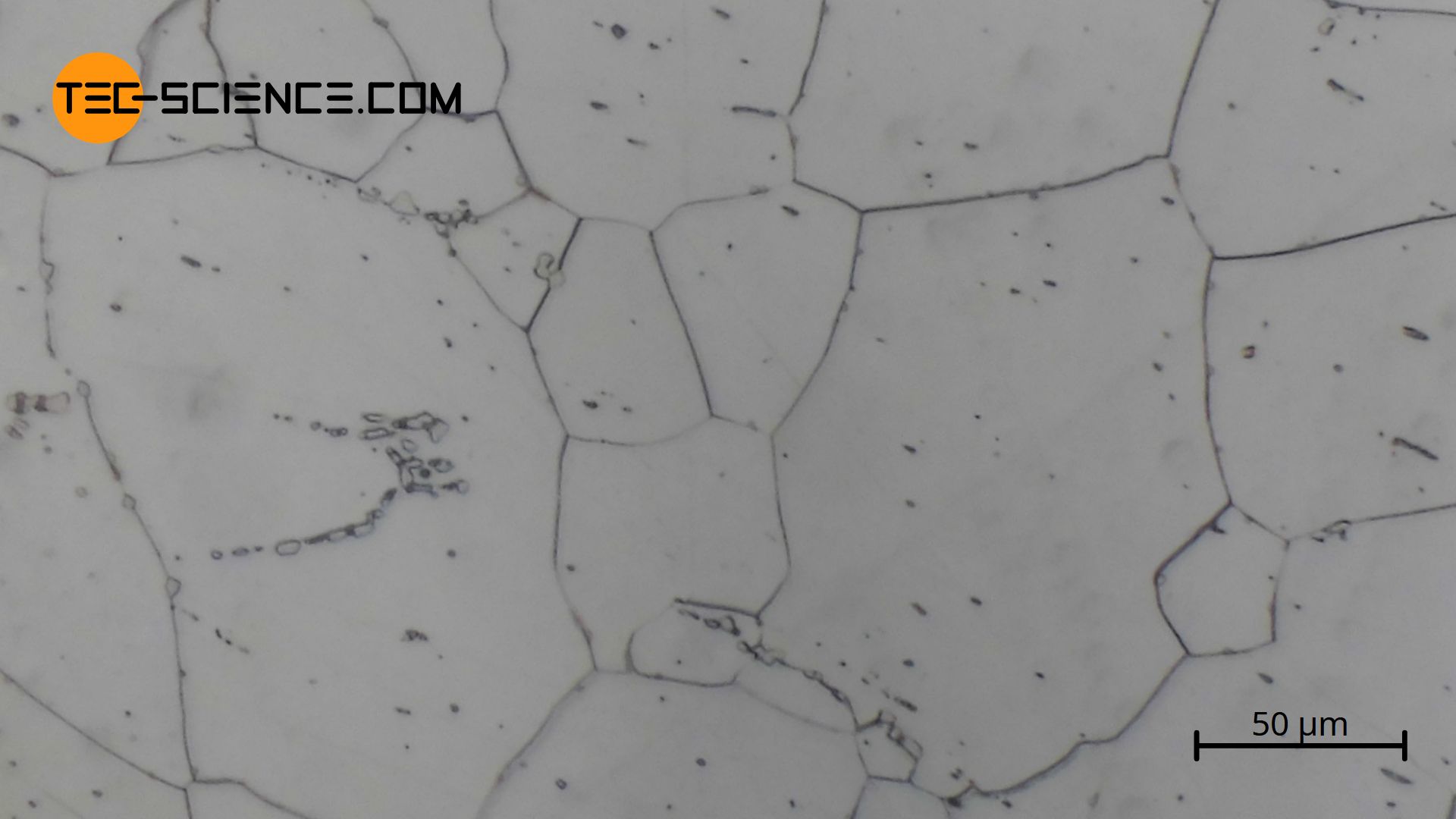
Now that the microstructural transformations of pure iron have been explained, the following article describes the phase transformations in the presence of carbon (steel) in more detail.
Microstructure formation of steel
In the previous section, the phase transformations of pure iron were examined in more detail. In addition to iron, however, steels also consist of carbon. This leads to a shift in the described phase transformations of the iron! How the carbon influences the phase transitions ist best explained by the corresponding phase diagram (state diagram).
The state diagram of the iron-carbon system is also called the iron-carbon phase diagram. Due to its complexity, the creation of the phase diagram on the basis of selected cooling curves will not be discussed. Furthermore, the iron-carbon diagram in the following sections is initially only considered up to a carbon content of around 2%, as only this range is relevant for steels. This area in the iron-carbon diagram is therefore also referred to as the steel part. Higher carbon concentrations are discussed in more detail in separate sections.
The steel part is the section of the iron-carbon phase diagram up to a carbon content of 2% relevant for steels!
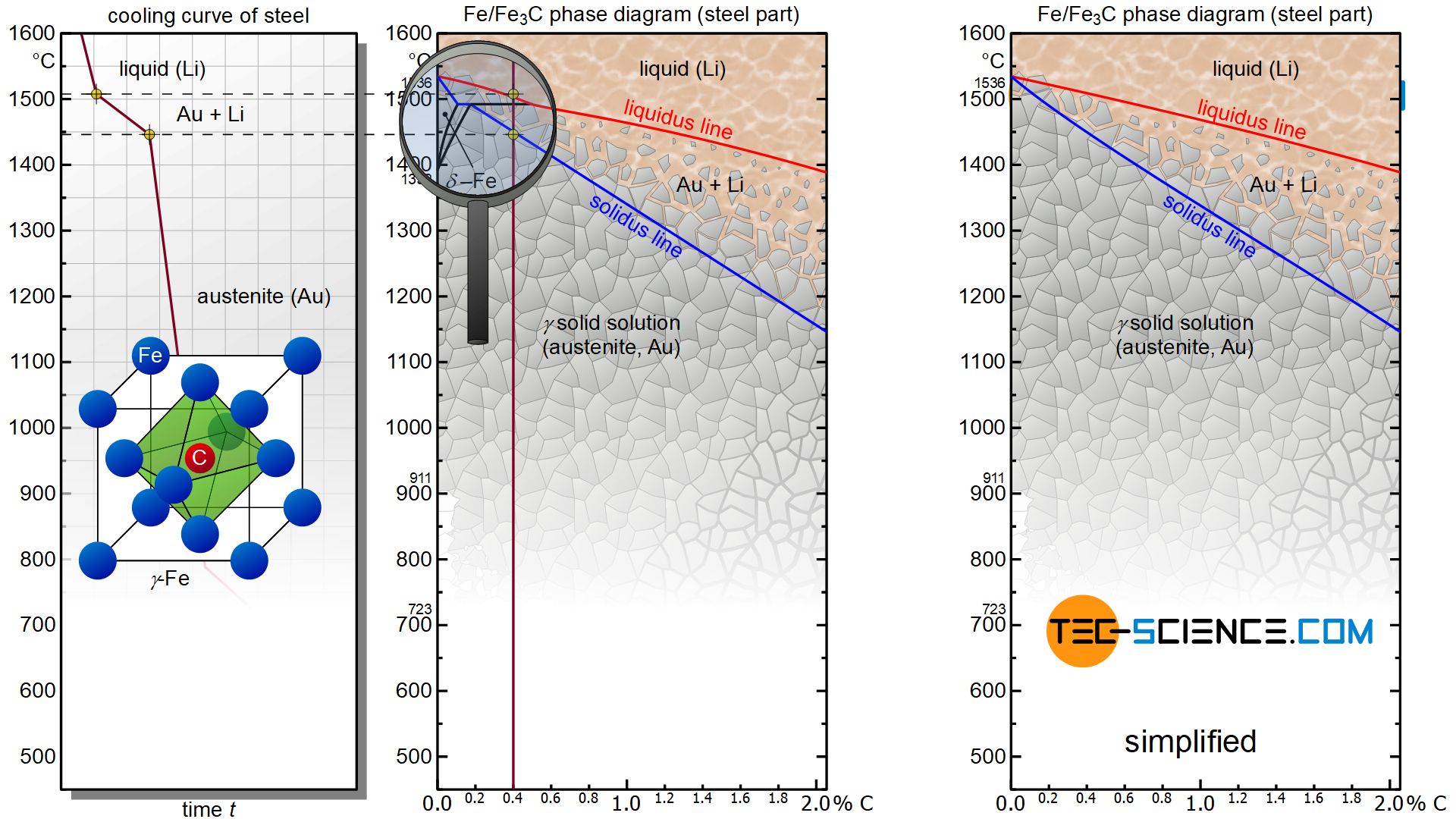
Carbon initially influences the solidification of the steel like a solid solution. The steel part of the phase diagram terefore has the typical lenticular two-phase region during solidification. The start of solidification is described by the liquidus line and the end of solidification by the solidus line. The microstructure is formed between these lines with a correspondingly slower cooling rate. The phase diagram shows that the solidification range shifts towards lower temperatures with increasing carbon content.
Carbon shifts the solidification range of the steel towards lower temperatures!
In addition, even small amounts of carbon (> 0.1%) completely suppress the body-centered cubic phase of \(\delta\)-iron. The steel then immediately crystallizes in the face-centered cubic lattice structure of \(\gamma\)-iron. Since the \(\delta\) phase has no technical significance anyway, the phase diagram is very often presented in simplified form without this phase region.
Steels behave during solidification like solid solutions in which the alloying element carbon is completely soluble in the host material iron.
The good solubility of carbon is due to the face-centered cubic lattice structure of \(\gamma\)-iron. The relatively small carbon atoms find their place in the free centers of the unit cells. In this case, it is a interstitial solid solution in which the carbon atom is embedded in the interstitials of the iron lattice. This face-centered cubic lattice structure of iron with carbon atoms embedded in it is also called austenite.
Austenite is the face-centered cubic lattice structure of \(\gamma\)-iron with carbon atoms embedded therein (solid solution)!
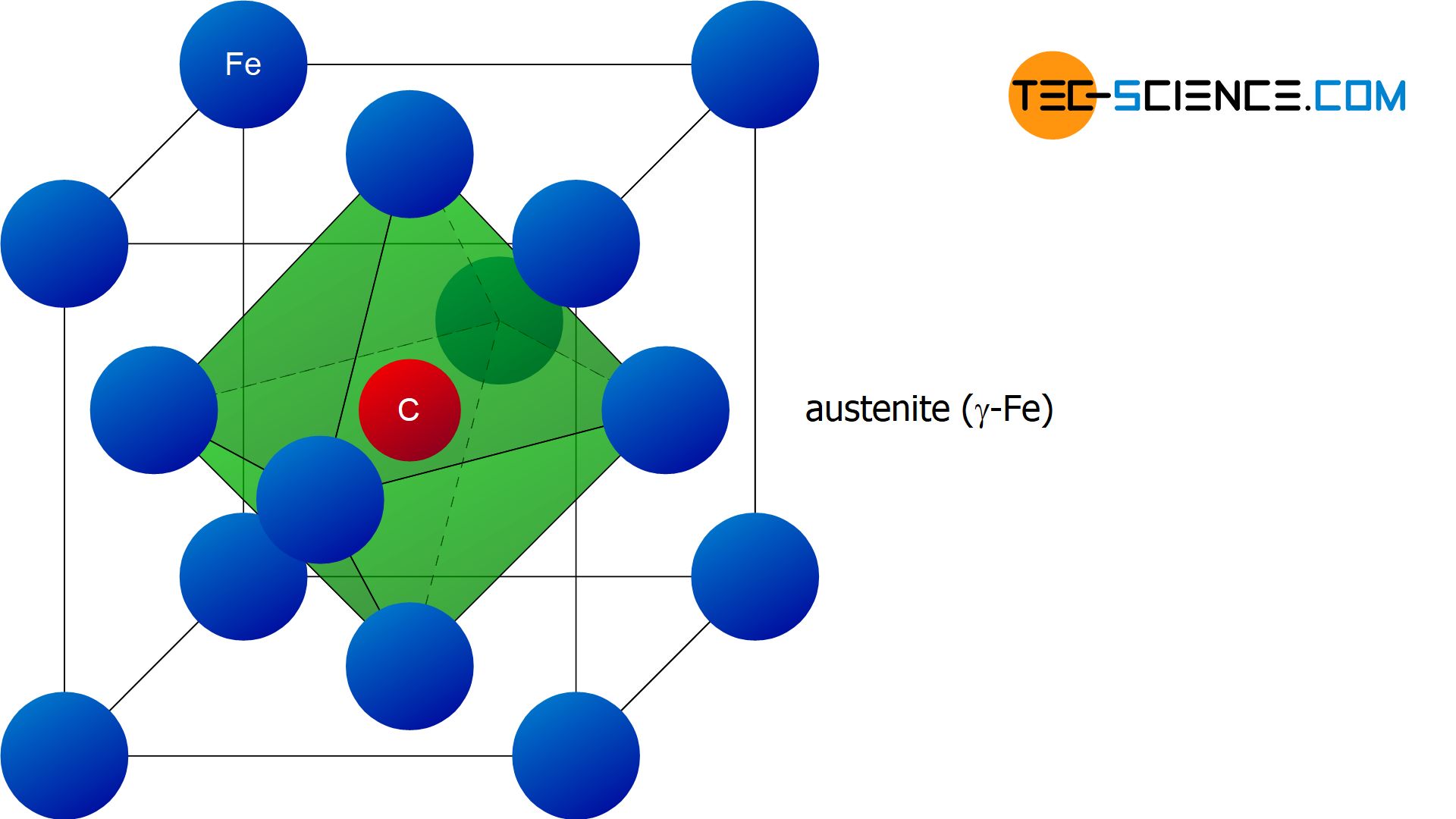
Accordingly, the two-phase region between the liquidus line and the solidus lines contains the phases melt (L) and austenite (A). In the two-phase region, the respective carbon concentrations of the two phases can be determined as usual by drawing a perpendicular line onto the concentration axis. The phase fractions are again determined by means of the lever rule.
In general, the same basic mechanisms take place during solidification of steels as for solid solutions. However, this only applies as long as the temperatures are sufficiently high and the iron is thus in the face-centered cubic state. Only then is the carbon completely soluble in the iron lattice and the alloy can be regarded as a solid solution.
The austenite phase only exists at sufficiently high temperatures as long as the iron is present in the face-centered cubic structure!
However, due to its allotropy, when the temperature drops, iron eventually changes its face-centered cubic structure and transforms into the body-centered cubic \(\alpha\)-iron. With decreasing temperature a further phase transformation is connected, which takes place now however in the already solidified microstructure! This conversion will be discussed in more detail in the next section.
Carbon precipitation (\(\gamma\)-\(\alpha\)-transformation)
Pure iron changes its face-centered cubic lattice structure of \(\gamma\)-iron when the temperature falls below 911 °C and changes to the body-centered cubic lattice structure of \(\alpha\)-iron. In principle, this lattice transformation also occurs in the presence of carbon, but at other temperatures!
As the carbon content increases, this so-called \(\gamma\)-\(\alpha\)-transformation is shifted towards lower temperatures. In addition, the carbon causes this lattice transformation to take place in a temperature range rather than in thermal arrest at a constant temperature. Only from a carbon content of 0.8 % does the \(\alpha\)-iron form again at constant temperature so that the polylines of the beginning and end of the \(\gamma\)-\(\alpha\)-conversion coincide in the phase diagram.
The presence of carbon shifts the \(\gamma\)-\(\alpha\)-transformation towards lower temperatures!
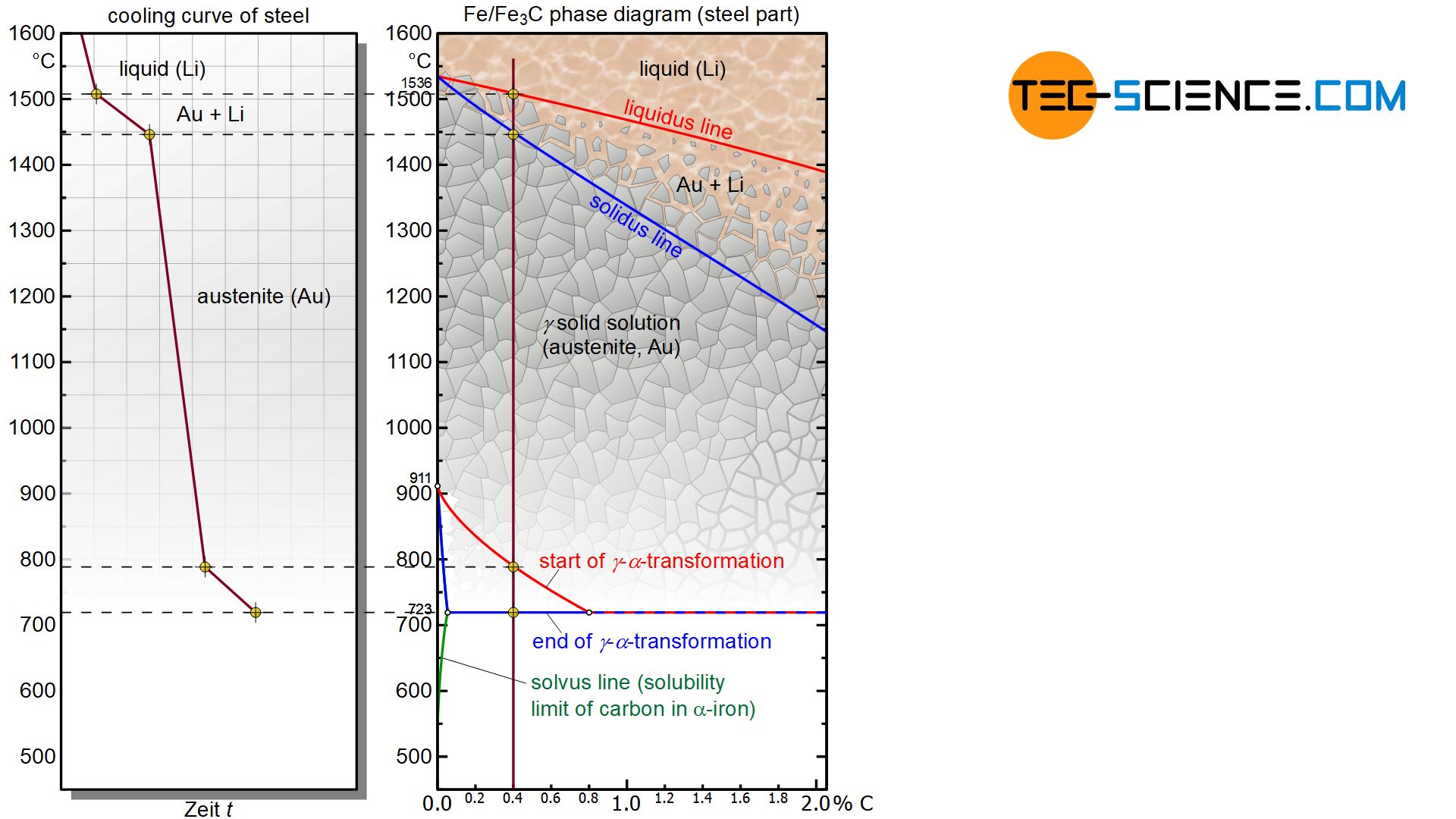
In contrast to the solid solution of \(\gamma\)-iron, the unit cell of the body-centered cubic lattice of \(\alpha\)-iron is already occupied by an iron atom in the center of the cube. \(\alpha\)-iron can therefore dissolve almost no carbon. The maximum solubility at 723 °C is only 0.02 % and even drops below 0.001 % at room temperature (the exact solubility limit is shown in the diagram with a green solvus line). To simplify matters, it is therefore assumed in the following that no carbon is soluble in the lattice of \(\alpha\)-iron.
The carbon atom previously embedded in the austenite is therefore “pressed out” of the lattice structure during the \(\gamma\)-\(\alpha\)-transformation. Thus, it is an almost carbon-free \(\alpha\)-iron lattice. In contrast to the carbon-containing face-centered cubic lattice of \(\gamma\)-iron, which was called austenite, the almost carbon-free body-centered cubic lattice of \(\alpha\)-iron is also called ferrite.
Ferrite is the almost carbon-free cubic space-centered lattice structure of \(\alpha\)-iron!
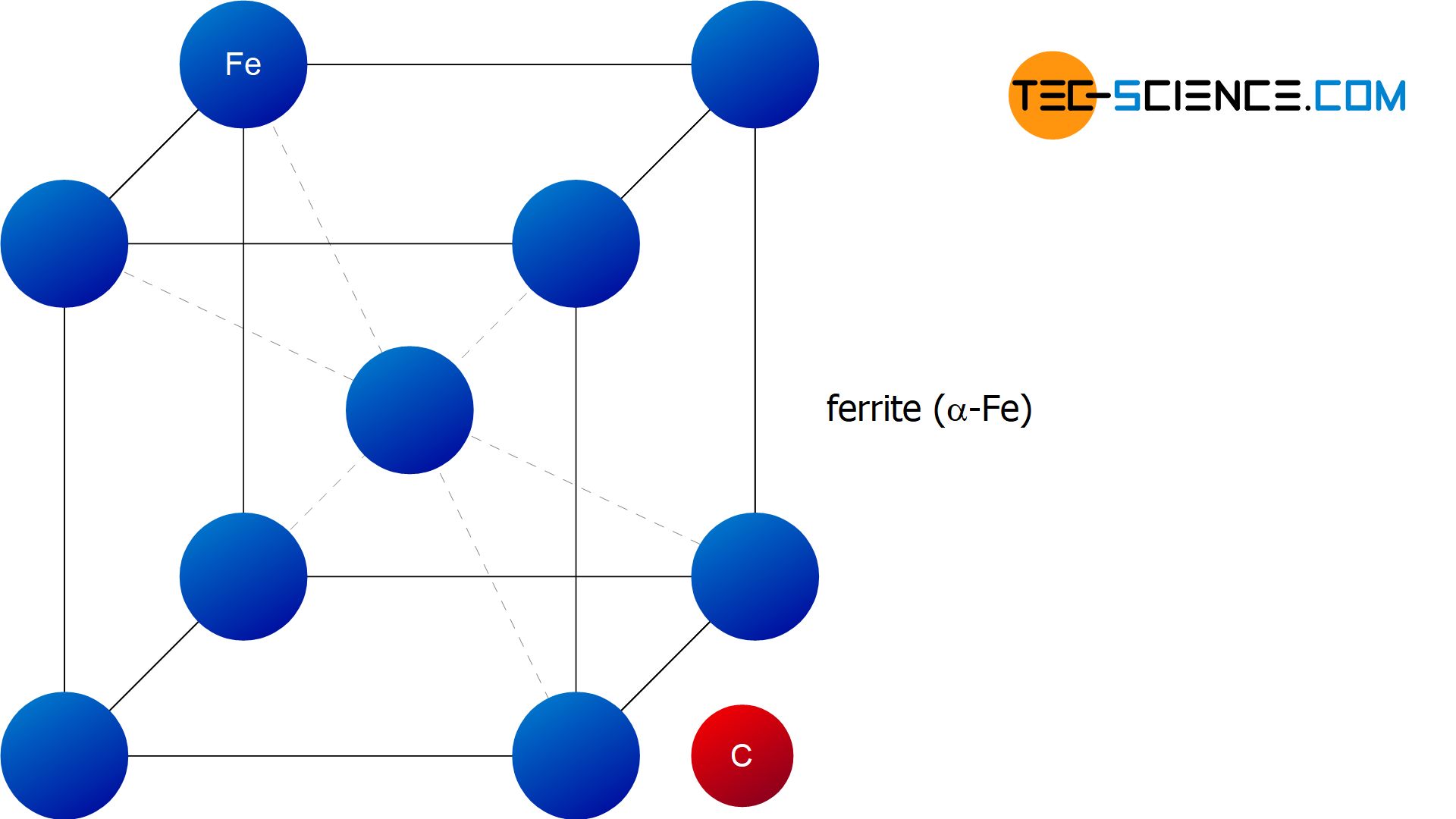
Stable system
During the \(\gamma\)-\(\alpha\)-conversion, the carbon that is no longer soluble in \(\alpha\)-iron can in principle precipitate from the lattice in two ways. With slow cooling and a relatively high carbon content, a sufficient number of carbon atoms can come together to form their own hexagonal lattice structure. In this lattice modification, carbon is also called graphite.
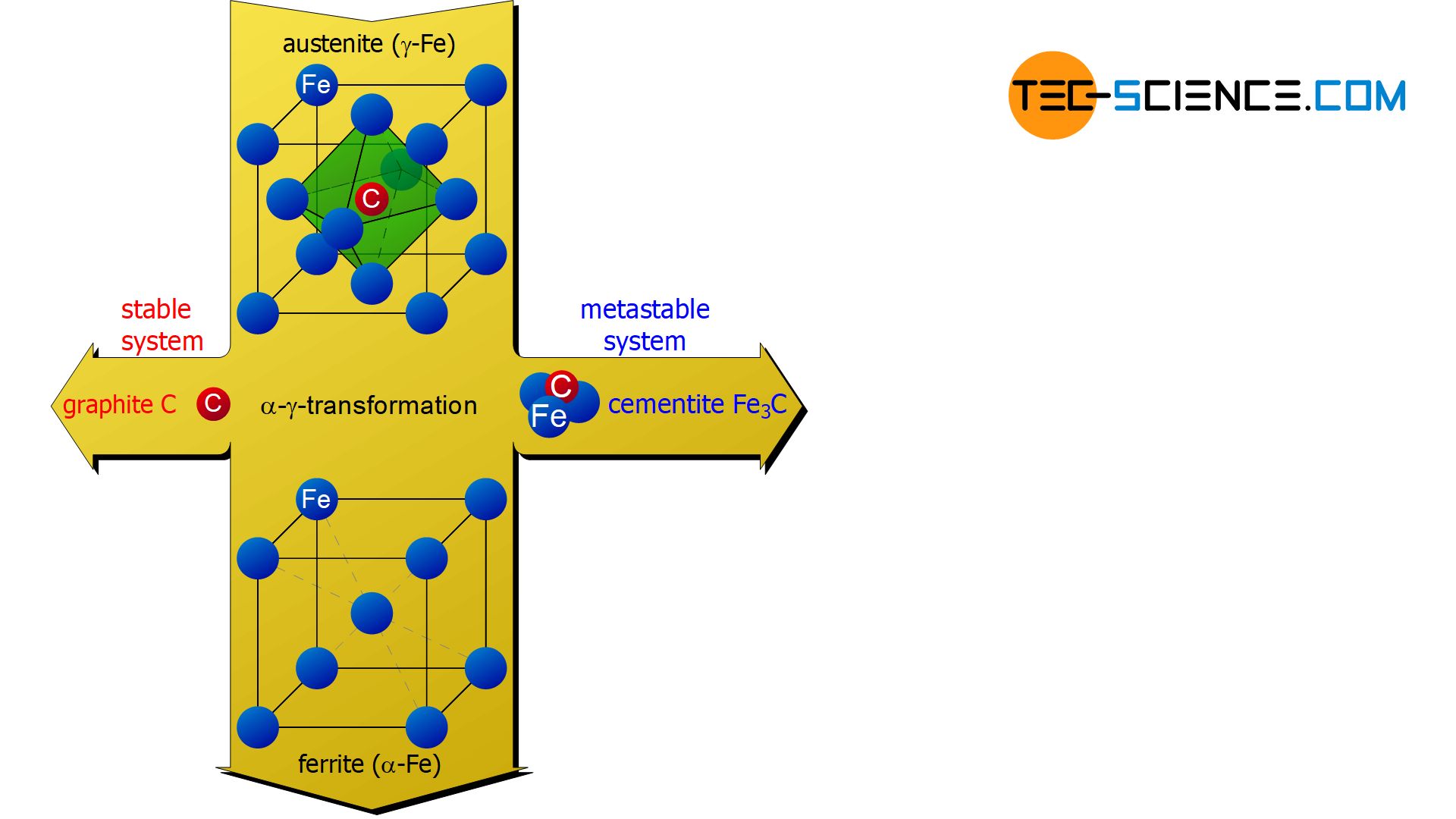
Such graphite precipitation is not only favoured by relatively slow cooling speeds but can also be specifically promoted by adding silicon. The precipitation of carbon in the form of graphite is also referred to as a stable system, since the carbon in this form can no longer decay further and is therefore stable in the thermodynamic sense.
A microstructure solidified according to the stable system basically consists of iron (Fe) and graphite (C). This applies in particular to cast iron!
Cast iron usually has a relatively high carbon content (> 2 %) and is therefore a typical representative of the stable system. However, some types of cast iron also solidify according to the metastable system described below. This applies in particular to steels.
Metastable system
If the solidified microstructure is no longer cooled relatively slowly but faster and only small amounts of carbon are present, the carbon atoms can no longer attach to a common graphite lattice structure. In this case, the precipitating carbon combines with three iron atoms to form the iron carbide compound Fe3C and forms a rhombohedral lattice structure. This intermediate (intermetallic) iron carbide compound is also called cementite.
Cementite is a relatively hard but brittle intermetallic compound consisting of three iron atoms and one carbon atom (Fe3C)!
As the name suggests, cementite is very hard and significantly responsible for the increase in hardness of the steel! Precipitation of cementite can not only be achieved through faster cooling but also by specific additives such as manganese. The precipitation of carbon in the form of cementite is also called a metastable system in the thermodynamic sense, since the iron carbide compound would decompose into the thermodynamically stable graphite form by diffusion processes at sufficiently high temperatures and sufficiently long annealing times.
In contrast to cast iron, steels generally have a relatively low carbon content (< 2 %) and are therefore typical representatives of the metastable system.
A microstructure solidified according to the metastable system basically consists of iron (Fe) and cementite (Fe3C). This applies in particular to steels!
Depending on the precipitation of carbon in the form of graphite or cementite, the polylines in the iron-carbon phase diagram differ slightly from one another (more on this in the article on cast iron). Since the metastable system with its cementite precipitation is particularly important for steels, only this metastable system will be discussed in more detail in the following articles.